Abstract
The use of next-generation sequencing technologies such as exome and genome sequencing in research and clinical care has transformed our understanding of the molecular architecture of genetic kidney diseases. Although the capability to identify and rigorously assess genetic variants and their relationship to disease has advanced considerably in the past decade, the curation of clinically relevant relationships between genes and specific phenotypes has received less attention, despite it underpinning accurate interpretation of genomic tests. Here, we discuss the need to accurately define gene–disease relationships in nephrology and provide a framework for appraising genetic and experimental evidence critically. We describe existing international programmes that provide expert curation of gene–disease relationships and discuss sources of discrepancy as well as efforts at harmonization. Further, we highlight the need for alignment of disease and phenotype terminology to ensure robust and reproducible curation of knowledge. These collective efforts to support evidence-based translation of genomic sequencing into practice across clinical, diagnostic and research settings are crucial for delivering the promise of precision medicine in nephrology, providing more patients with timely diagnoses, accurate prognostic information and access to targeted treatments.
Key points
-
Genomic sequencing technologies are transforming our understanding of genetic kidney disease.
-
Evidence-based frameworks, such as the American College of Medical Genetics and Genomics and Association for Molecular Pathology guidelines, are crucial to the appraisal of individual genetic variants and disease.
-
Curation of clinically relevant relationships between genes and specific phenotypes underpins accurate interpretation of genomic tests and, in turn, the ability to provide accurate and timely molecular diagnosis to patients, informing prognosis, recurrence risk counselling and, increasingly, access to precision treatments.
-
Several collaborative initiatives are undertaking gene–disease curation in genetic kidney disease within the international Gene Curation Coalition, including ClinGen, Genomics England PanelApp, Online Mendelian Inheritance in Man, Orphanet and PanelApp Australia.
-
The ClinGen Kidney Domain Working Group expert panels use the ClinGen semi-quantitative framework to systematically appraise gene–disease relationships in nephrology. Classifications are determined collaboratively between disease and curation experts on the basis of critical appraisal of human and experimental data and are publicly available.
-
As the molecular basis of genetic kidney diseases is elucidated, disease nomenclature will need to evolve; a two-tiered, dyadic naming model (core disease name–gene name), augmented with additional terms as applicable, can be applied consistently for most Mendelian kidney diseases.
This is a preview of subscription content, access via your institution
Access options
Access Nature and 54 other Nature Portfolio journals
Get Nature+, our best-value online-access subscription
24,99 € / 30 days
cancel any time
Subscribe to this journal
Receive 12 print issues and online access
209,00 € per year
only 17,42 € per issue
Buy this article
- Purchase on SpringerLink
- Instant access to full article PDF
Prices may be subject to local taxes which are calculated during checkout
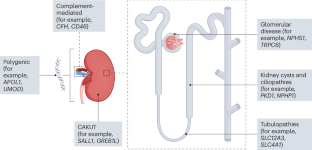
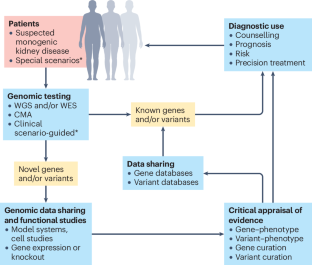
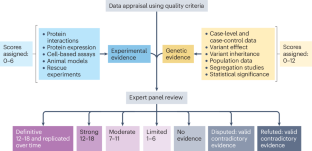
Similar content being viewed by others
References
Chronic Kidney Disease Collaboration, Global Burden of Disease. Global, regional, and national burden of chronic kidney disease, 1990–2017: a systematic analysis for the Global Burden of Disease Study 2017. Lancet 395, 709–733 (2020).
Chadban, S. J. et al. Prevalence of kidney damage in Australian adults: The AusDiab kidney study. J. Am. Soc. Nephrol. 14, S131–S138 (2003).
Coresh, J. et al. Prevalence of chronic kidney disease in the United States. JAMA 298, 2038–2047 (2007).
Fletcher, J., McDonald, S., Alexander, S. I. & Australian & New Zealand Pediatric Nephrology Association. Prevalence of genetic renal disease in children. Pediatr. Nephrol. 28, 251–256 (2013).
Hildebrandt, F. Decade in review-genetics of kidney diseases: genetic dissection of kidney disorders. Nat. Rev. Nephrol. 11, 635–636 (2015).
Vivante, A. & Hildebrandt, F. Exploring the genetic basis of early-onset chronic kidney disease. Nat. Rev. Nephrol. 12, 133–146 (2016).
Cocchi, E., Nestor, J. G. & Gharavi, A. G. Clinical genetic screening in adult patients with kidney disease. Clin. J. Am. Soc. Nephrol. 15, 1497–1510 (2020).
Mallett, A. et al. The prevalence and epidemiology of genetic renal disease amongst adults with chronic kidney disease in Australia. Orphanet J. Rare Dis. 9, 98 (2014).
Schrezenmeier, E. et al. The underestimated burden of monogenic kidney disease in adults waitlisted for kidney transplantation. Genet. Med. 23, 1219–1224 (2021).
Snoek, R. et al. Genetics-first approach improves diagnostics of ESKD patients <50 years old. Nephrol. Dial. Transpl. 37, 349–357 (2022).
Sedor, J. R. APOL1 kidney disease: discovery to targeted therapy in 10 years. Clin. J. Am. Soc. Nephrol. 19, 126–128 (2023).
Cornec-Le Gall, E., Alam, A. & Perrone, R. D. Autosomal dominant polycystic kidney disease. Lancet 393, 919–935 (2019).
Mallett, A. J., Knoers, N., Sayer, J. & Stark, Z. Clinical versus research genomics in kidney disease. Nat. Rev. Nephrol. 17, 570–571 (2021).
Groopman, E. E. et al. Diagnostic utility of exome sequencing for kidney disease. N. Engl. J. Med. 380, 142–151 (2019).
Jayasinghe, K. et al. Clinical impact of genomic testing in patients with suspected monogenic kidney disease. Genet. Med. 23, 183–191 (2021).
Tanudisastro, H. A. et al. Australia and New Zealand renal gene panel testing in routine clinical practice of 542 families. NPJ Genom. Med. 6, 20 (2021).
Dahl, N. K. et al. The clinical utility of genetic testing in the diagnosis and management of adults with chronic kidney disease. J. Am. Soc. Nephrol. 34, 2039–2050 (2023).
Claus, L. R., Snoek, R., Knoers, N. & van Eerde, A. M. Review of genetic testing in kidney disease patients: Diagnostic yield of single nucleotide variants and copy number variations evaluated across and within kidney phenotype groups. Am. J. Med. Genet. C. Semin. Med. Genet. 190, 358–376 (2022).
Jayasinghe, K. et al. Cost-effectiveness of targeted exome analysis as a diagnostic test in glomerular diseases. Kidney Int. Rep. 6, 2850–2861 (2021).
Wu, Y. et al. Genomic testing for suspected monogenic kidney disease in children and adults: a health economic evaluation. Genet. Med. 25, 100942 (2023).
Becherucci, F. et al. A clinical workflow for cost-saving high-rate diagnosis of genetic kidney diseases. J. Am. Soc. Nephrol. 34, 706–720 (2023).
Garrelfs, S. F. et al. Lumasiran, an RNAi therapeutic for primary hyperoxaluria type 1. N. Engl. J. Med. 384, 1216–1226 (2021).
Germain, D. P. et al. An expert consensus on practical clinical recommendations and guidance for patients with classic Fabry disease. Mol. Genet. Metab. 137, 49–61 (2022).
Heather, J. M. & Chain, B. The sequence of sequencers: the history of sequencing DNA. Genomics 107, 1–8 (2016).
Shendure, J. et al. DNA sequencing at 40: past, present and future. Nature 550, 345–353 (2017).
Lander, E. S. et al. Initial sequencing and analysis of the human genome. Nature 409, 860–921 (2001).
Venter, J. C. et al. The sequence of the human genome. Science 291, 1304–1351 (2001).
Stark, Z. et al. Integrating genomics into healthcare: a global responsibility. Am. J. Hum. Genet. 104, 13–20 (2019).
Gilissen, C., Hoischen, A., Brunner, H. G. & Veltman, J. A. Unlocking Mendelian disease using exome sequencing. Genome Biol. 12, 228 (2011).
Richards, S. et al. Standards and guidelines for the interpretation of sequence variants: a joint consensus recommendation of the American College of Medical Genetics and Genomics and the Association for Molecular Pathology. Genet. Med. 17, 405–424 (2015).
Rehm, H. L. et al. GA4GH: International policies and standards for data sharing across genomic research and healthcare. Cell Genom 1, 100029 (2021).
Austin-Tse, C. A. et al. Best practices for the interpretation and reporting of clinical whole genome sequencing. NPJ Genom. Med. 7, 27 (2022).
Stark, Z. et al. Scaling national and international improvement in virtual gene panel curation via a collaborative approach to discordance resolution. Am. J. Hum. Genet. 108, 1551–1557 (2021).
Yuskaitis, C. J., Sheidley, B. R. & Poduri, A. Variability among next-generation sequencing panels for early-life epilepsies. JAMA Pediatr. 172, 779–780 (2018).
Ghosh, R. et al. Updated recommendation for the benign stand-alone ACMG/AMP criterion. Hum. Mutat. 39, 1525–1530 (2018).
Abou Tayoun, A. N. et al. Recommendations for interpreting the loss of function PVS1 ACMG/AMP variant criterion. Hum. Mutat. 39, 1517–1524 (2018).
Oza, A. M. et al. Expert specification of the ACMG/AMP variant interpretation guidelines for genetic hearing loss. Hum. Mutat. 39, 1593–1613 (2018).
Patel, M. J. et al. Disease-specific ACMG/AMP guidelines improve sequence variant interpretation for hearing loss. Genet. Med. 23, 2208–2212 (2021).
Kelly, M. A. et al. Adaptation and validation of the ACMG/AMP variant classification framework for MYH7-associated inherited cardiomyopathies: recommendations by ClinGen’s Inherited Cardiomyopathy Expert Panel. Genet. Med. 20, 351–359 (2018).
Fowler, D. M. & Rehm, H. L. Will variants of uncertain significance still exist in 2030? Am. J. Hum. Genet. 111, 5–10 (2024).
Rehm, H. L. et al. The landscape of reported VUS in multi-gene panel and genomic testing: time for a change. Genet. Med. 25, 100947 (2023).
Gunter, C. & Green, E. D. To boldly go: unpacking the NHGRI’s bold predictions for human genomics by 2030. Am. J. Hum. Genet. 110, 1829–1831 (2023).
Dai, P. et al. Recommendations for next generation sequencing data reanalysis of unsolved cases with suspected Mendelian disorders: a systematic review and meta-analysis. Genet. Med. 24, 1618–1629 (2022).
Robertson, A. J. et al. Re-analysis of genomic data: an overview of the mechanisms and complexities of clinical adoption. Genet. Med. 24, 798–810 (2022).
Fehlberg, Z., Stark, Z. & Best, S. Reanalysis of genomic data, how do we do it now and what if we automate it? A qualitative study. Eur J Hum Genet. 32, 521–528 (2024).
Bamshad, M. J., Nickerson, D. A. & Chong, J. X. Mendelian gene discovery: fast and furious with no end in sight. Am. J. Hum. Genet. 105, 448–455 (2019).
Baxter, S. M. et al. Centers for Mendelian Genomics: a decade of facilitating gene discovery. Genet. Med. 24, 784–797 (2022).
Barker, D. F. et al. Identification of mutations in the COL4A5 collagen gene in Alport syndrome. Science 248, 1224–1227 (1990).
Li, A. S., Ingham, J. F. & Lennon, R. Genetic disorders of the glomerular filtration barrier. Clin. J. Am. Soc. Nephrol. 15, 1818–1828 (2020).
The European Polycystic Kidney Disease Consortium. The polycystic kidney disease 1 gene encodes a 14 kb transcript and lies within a duplicated region on chromosome 16. Cell 78, 725 (1994).
Rossetti, S. et al. Incompletely penetrant PKD1 alleles suggest a role for gene dosage in cyst initiation in polycystic kidney disease. Kidney Int. 75, 848–855 (2009).
Durkie, M., Chong, J., Valluru, M. K., Harris, P. C. & Ong, A. C. M. Biallelic inheritance of hypomorphic PKD1 variants is highly prevalent in very early onset polycystic kidney disease. Genet. Med. 23, 689–697 (2021).
Perrault, I. et al. Mainzer-Saldino syndrome is a ciliopathy caused by IFT140 mutations. Am. J. Hum. Genet. 90, 864–870 (2012).
Senum, S. R. et al. Monoallelic IFT140 pathogenic variants are an important cause of the autosomal dominant polycystic kidney-spectrum phenotype. Am. J. Hum. Genet. 109, 136–156 (2022).
Chen, S. et al. A genomic mutational constraint map using variation in 76,156 human genomes. Nature 625, 92–100. (2023).
Hoskins, B. E. et al. Transcription factor SIX5 is mutated in patients with branchio-oto-renal syndrome. Am. J. Hum. Genet. 80, 800–804 (2007).
Hwang, D. Y. et al. Mutations in 12 known dominant disease-causing genes clarify many congenital anomalies of the kidney and urinary tract. Kidney Int. 85, 1429–1433 (2014).
Krug, P. et al. Mutation screening of the EYA1, SIX1, and SIX5 genes in a large cohort of patients harboring branchio-oto-renal syndrome calls into question the pathogenic role of SIX5 mutations. Hum. Mutat. 32, 183–190 (2011).
Klesert, T. R. et al. Mice deficient in Six5 develop cataracts: implications for myotonic dystrophy. Nat. Genet. 25, 105–109 (2000).
Sarkar, P. S. et al. Heterozygous loss of Six5 in mice is sufficient to cause ocular cataracts. Nat. Genet. 25, 110–114 (2000).
KDIGO Conference Participants. Genetics in chronic kidney disease: conclusions from a Kidney Disease: Improving Global Outcomes (KDIGO) Controversies Conference. Kidney Int. 101, 1126–1141 (2022).
Cornec-Le Gall, E. et al. The PROPKD Score: a new algorithm to predict renal survival in autosomal dominant polycystic kidney disease. J. Am. Soc. Nephrol. 27, 942–951 (2016).
Hwang, Y. H. et al. Refining genotype-phenotype correlation in autosomal dominant polycystic kidney disease. J. Am. Soc. Nephrol. 27, 1861–1868 (2016).
Gibson, J. T. et al. Genotype-phenotype correlations for COL4A3–COL4A5 variants resulting in Gly substitutions in Alport syndrome. Sci. Rep. 12, 2722 (2022).
Bekheirnia, M. R. et al. Genotype-phenotype correlation in X-linked Alport syndrome. J. Am. Soc. Nephrol. 21, 876–883 (2010).
Reeders, S. T. et al. A highly polymorphic DNA marker linked to adult polycystic kidney disease on chromosome 16. Nature 317, 542–544 (1985).
The International Polycystic Kidney Disease Consortium. Polycystic kidney disease: the complete structure of the PKD1 gene and its protein. Cell 81, 289–298 (1995).
Gattone, V. H. 2nd, Wang, X., Harris, P. C. & Torres, V. E. Inhibition of renal cystic disease development and progression by a vasopressin V2 receptor antagonist. Nat. Med. 9, 1323–1326 (2003).
Yamamura, Y. et al. OPC-41061, a highly potent human vasopressin V2-receptor antagonist: pharmacological profile and aquaretic effect by single and multiple oral dosing in rats. J. Pharmacol. Exp. Ther. 287, 860–867 (1998).
Torres, V. E. et al. Tolvaptan in patients with autosomal dominant polycystic kidney disease. N. Engl. J. Med. 367, 2407–2418 (2012).
Torres, V. E. et al. Tolvaptan in later-stage autosomal dominant polycystic kidney disease. N. Engl. J. Med. 377, 1930–1942 (2017).
Chebib, F. T. et al. A practical guide for treatment of rapidly progressive ADPKD with tolvaptan. J. Am. Soc. Nephrol. 29, 2458–2470 (2018).
Besbas, N. et al. A classification of hemolytic uremic syndrome and thrombotic thrombocytopenic purpura and related disorders. Kidney Int. 70, 423–431 (2006).
Kavanagh, D., Richards, A. & Atkinson, J. Complement regulatory genes and hemolytic uremic syndromes. Annu. Rev. Med. 59, 293–309 (2008).
Thomas, T. C. et al. Inhibition of complement activity by humanized anti-C5 antibody and single-chain Fv. Mol. Immunol. 33, 1389–1401 (1996).
Nurnberger, J. et al. Eculizumab for atypical hemolytic-uremic syndrome. N. Engl. J. Med. 360, 542–544 (2009).
Gruppo, R. A. & Rother, R. P. Eculizumab for congenital atypical hemolytic-uremic syndrome. N. Engl. J. Med. 360, 544–546 (2009).
Mache, C. J. et al. Complement inhibitor eculizumab in atypical hemolytic uremic syndrome. Clin. J. Am. Soc. Nephrol. 4, 1312–1316 (2009).
Schmidtko, J., Peine, S., El-Housseini, Y., Pascual, M. & Meier, P. Treatment of atypical hemolytic uremic syndrome and thrombotic microangiopathies: a focus on eculizumab. Am. J. Kidney Dis. 61, 289–299 (2013).
Kuzmuk, V. et al. A small molecule chaperone rescues keratin-8 mediated trafficking of misfolded podocin to correct genetic nephrotic syndrome. Kidney Int. 105, 744–758 (2023).
Ding, W. Y. et al. Adeno-associated virus gene therapy prevents progression of kidney disease in genetic models of nephrotic syndrome. Sci. Transl. Med. 15, eabc8226 (2023).
Dvela-Levitt, M. et al. Small molecule targets TMED9 and promotes lysosomal degradation to reverse proteinopathy. Cell 178, 521–535 e523 (2019).
Egbuna, O. et al. Inaxaplin for proteinuric kidney disease in persons with two APOL1 variants. N. Engl. J. Med. 388, 969–979 (2023).
Boute, N. et al. NPHS2, encoding the glomerular protein podocin, is mutated in autosomal recessive steroid-resistant nephrotic syndrome. Nat. Genet. 24, 349–354 (2000).
Kirby, A. et al. Mutations causing medullary cystic kidney disease type 1 lie in a large VNTR in MUC1 missed by massively parallel sequencing. Nat. Genet. 45, 299–303 (2013).
Genovese, G. et al. Association of trypanolytic ApoL1 variants with kidney disease in African Americans. Science 329, 841–845 (2010).
MacArthur, D. G. et al. Guidelines for investigating causality of sequence variants in human disease. Nature 508, 469–476 (2014).
Strande, N. T. et al. Evaluating the clinical validity of gene-disease associations: an evidence-based framework developed by the Clinical Genome Resource. Am. J. Hum. Genet. 100, 895–906 (2017).
Boycott, K. M., Azzariti, D. R., Hamosh, A. & Rehm, H. L. Seven years since the launch of the Matchmaker Exchange: the evolution of genomic matchmaking. Hum. Mutat. 43, 659–667 (2022).
Martin, A. R. et al. PanelApp crowdsources expert knowledge to establish consensus diagnostic gene panels. Nat. Genet. 51, 1560–1565 (2019).
DiStefano, M. T. et al. The Gene Curation Coalition: a global effort to harmonize gene-disease evidence resources. Genet. Med. 24, 1732–1742 (2022).
Roberts, A. M. et al. Towards robust clinical genome interpretation: developing a consistent terminology to characterize Mendelian disease-gene relationships — allelic requirement, inheritance modes and disease mechanisms. Genet. Med. 26, 101029 (2023).
Tudini, E. et al. Shariant platform: enabling evidence sharing across Australian clinical genetic-testing laboratories to support variant interpretation. Am. J. Hum. Genet. 109, 1960–1973 (2022).
Thaxton, C. et al. Lumping versus splitting: how to approach defining a disease to enable accurate genomic curation. Cell Genom. 2, 100131 (2022).
Milko, L. V. et al. Development of clinical domain working groups for the clinical genome resource (ClinGen): lessons learned and plans for the future. Genet. Med. 21, 987–993 (2019).
Welch, C. L. et al. Defining the clinical validity of genes reported to cause pulmonary arterial hypertension. Genet. Med. 25, 100925 (2023).
Riggs, E. R. et al. Clinical validity assessment of genes frequently tested on intellectual disability/autism sequencing panels. Genet. Med. 24, 1899–1908 (2022).
Walsh, R. et al. Evaluation of gene validity for CPVT and short QT syndrome in sudden arrhythmic death. Eur. Heart J. 43, 1500–1510 (2022).
Jordan, E. et al. Evidence-based assessment of genes in dilated cardiomyopathy. Circulation 144, 7–19 (2021).
Hosseini, S. M. et al. Reappraisal of reported genes for sudden arrhythmic death: evidence-based evaluation of gene validity for Brugada Syndrome. Circulation 138, 1195–1205 (2018).
Rehm, H. L. et al. ClinGen — the Clinical Genome Resource. N. Engl. J. Med. 372, 2235–2242 (2015).
Rasmussen, S. A., Hamosh, A. & Curators, O. What’s in a name? Issues to consider when naming Mendelian disorders. Genet. Med. 22, 1573–1575 (2020).
Biesecker, L. G. et al. A dyadic approach to the delineation of diagnostic entities in clinical genomics. Am. J. Hum. Genet. 108, 8–15 (2021).
World Health Organization. International Classification of Diseases, Eleventh Revision (ICD-11) Vol. 2024 (2019).
Vasilevsky, N. A. et al. Mondo: unifying diseases for the world, by the world. Preprint at medRxiv https://doi.org/10.1101/2022.04.13.22273750 (2022).
Acknowledgements
The authors acknowledge the support and contributions of the ClinGen Kidney Disease Clinical Domain Working Group and its associated Gene Curation and Variant Curation Expert Panels. A.J.M. is supported by a Queensland Health Advancing Clinical Research Fellowship. A.B.B. is supported by the ClinGen NIH grant U24 HG006834 (to Broad/Geisinger).
Author information
Authors and Affiliations
Contributions
All authors researched data for the article, made substantial contributions to discussions of the content and reviewed or edited the manuscript before submission. Z.S., A.B.B. and A.J.M. wrote the article.
Corresponding authors
Ethics declarations
Competing interests
The authors declare no competing interests.
Peer review
Peer review information
Nature Reviews Nephrology thanks Anna Köttgen and the other, anonymous, reviewer(s) for their contribution to the peer review of this work.
Additional information
Publisher’s note Springer Nature remains neutral with regard to jurisdictional claims in published maps and institutional affiliations.
Glossary
- Expressivity
-
Describes the degree to which different features of a disease manifest in affected individuals.
- Penetrance
-
Describes the likelihood that an individual with a disease-causing gene variant will manifest clinical features of the disease.
Rights and permissions
Springer Nature or its licensor (e.g. a society or other partner) holds exclusive rights to this article under a publishing agreement with the author(s) or other rightsholder(s); author self-archiving of the accepted manuscript version of this article is solely governed by the terms of such publishing agreement and applicable law.
About this article
Cite this article
Stark, Z., Byrne, A.B., Sampson, M.G. et al. A guide to gene–disease relationships in nephrology. Nat Rev Nephrol (2024). https://doi.org/10.1038/s41581-024-00900-7
Accepted:
Published:
DOI: https://doi.org/10.1038/s41581-024-00900-7