Abstract
Molecular nitrogen (N2) is cheap and widely available, but its unreactive nature is a challenge when attempting to functionalize it under mild conditions with other widely available substrates (such as carbon monoxide, CO) to produce value-added compounds. Biological N2 fixation can do this, but the industrial Haber–Bosch process for ammonia production operates under harsh conditions (450 degrees Celsius and 300 bar), even though both processes are thought to involve multimetallic catalytic sites1,2. And although molecular complexes capable of binding and even reducing N2 under mild conditions are known, with co-operativity between metal centres considered crucial for the N2 reduction step1,2,3,4,5,6,7,8,9,10,11,12,13,14, the multimetallic species involved are usually not well defined, and further transformation of N2-binding complexes to achieve N–H or N–C bond formation is rare2,6,8,10,15,16. Haber noted17, before an iron-based catalyst was adopted for the industrial Haber–Bosch process, that uranium and uranium nitride materials are very effective heterogeneous catalysts for ammonia production from N2. However, few examples of uranium complexes binding N2 are known18,19,20,21,22, and soluble uranium complexes capable of transforming N2 into ammonia or organonitrogen compounds have not yet been identified. Here we report the four-electron reduction of N2 under ambient conditions by a fully characterized complex with two Uiii ions and three K+ centres held together by a nitride group and a flexible metalloligand framework. The addition of H2 and/or protons, or CO to the resulting complex results in the complete cleavage of N2 with concomitant N2 functionalization through N–H or N–C bond-forming reactions. These observations establish that a molecular uranium complex can promote the stoichiometric transformation of N2 into NH3 or cyanate, and that a flexible, electron-rich, multimetallic, nitride-bridged core unit is a promising starting point for the design of molecular complexes capable of cleaving and functionalizing N2 under mild conditions.
This is a preview of subscription content, access via your institution
Access options
Access Nature and 54 other Nature Portfolio journals
Get Nature+, our best-value online-access subscription
24,99 € / 30 days
cancel any time
Subscribe to this journal
Receive 51 print issues and online access
199,00 € per year
only 3,90 € per issue
Buy this article
- Purchase on SpringerLink
- Instant access to full article PDF
Prices may be subject to local taxes which are calculated during checkout
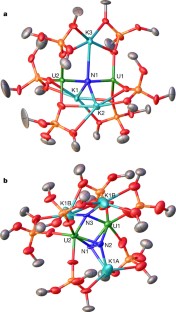
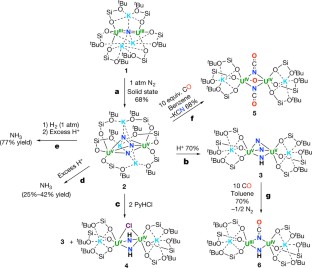
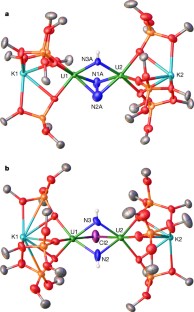
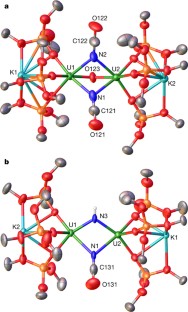
Similar content being viewed by others
References
MacKay, B. A. & Fryzuk, M. D. Dinitrogen coordination chemistry: on the biomimetic borderlands. Chem. Rev. 104, 385–401 (2004)
MacLeod, K. C. & Holland, P. L. Recent developments in the homogeneous reduction of dinitrogen by molybdenum and iron. Nat. Chem. 5, 559–565 (2013)
Gambarotta, S. & Scott, J. Multimetallic cooperative activation of N2 . Angew. Chem. Int. Edn Engl. 43, 5298–5308 (2004)
McWilliams, S. F. & Holland, P. L. Dinitrogen binding and cleavage by multinuclear iron complexes. Acc. Chem. Res. 48, 2059–2065 (2015)
Laplaza, C. E. & Cummins, C. C. Dinitrogen cleavage by a three-coordinate molybdenum(III). Science 268, 861–863 (1995)
Ohki, Y. & Fryzuk, M. D. Dinitrogen activation by group 4 metal complexes. Angew. Chem. Int. Edn Engl. 46, 3180–3183 (2007)
Pool, J. A., Lobkovsky, E. & Chirik, P. J. Hydrogenation and cleavage of dinitrogen to ammonia with a zirconium complex. Nature 427, 527–530 (2004)
Rodriguez, M. M., Bill, E., Brennessel, W. W. & Holland, P. L. N2 reduction and hydrogenation to ammonia by a molecular iron-potassium complex. Science 334, 780–783 (2011)
Fryzuk, M. D., Love, J. B., Rettig, S. J. & Young, V. G. Transformation of coordinated dinitrogen by reaction with dihydrogen and primary silanes. Science 275, 1445–1447 (1997)
Knobloch, D. J., Lobkovsky, E. & Chirik, P. J. Dinitrogen cleavage and functionalization by carbon monoxide promoted by a hafnium complex. Nat. Chem. 2, 30–35 (2010)
Arashiba, K., Miyake, Y. & Nishibayashi, Y. A molybdenum complex bearing PNP-type pincer ligands leads to the catalytic reduction of dinitrogen into ammonia. Nat. Chem. 3, 120–125 (2011)
Evans, W. J. et al. Isolation of dysprosium and yttrium complexes of a three-electron reduction product in the activation of dinitrogen, the (N2)3- radical. J. Am. Chem. Soc. 131, 11195–11202 (2009)
Lee, Y. et al. Dinitrogen activation upon reduction of a triiron(II) complex. Angew. Chem. Int. Edn Engl. 54, 1499–1503 (2015)
Hirotsu, M., Fontaine, P. P., Zavalij, P. Y. & Sita, L. R. Extreme N≡N bond elongation and facile N-atom functionalization reactions within two structurally versatile new families of group 4 bimetallic “side-on-bridged” dinitrogen complexes for zirconium and hafnium. J. Am. Chem. Soc. 129, 12690–12692 (2007)
Spencer, L. P., MacKay, B. A., Patrick, B. O. & Fryzuk, M. D. Inner-sphere two-electron reduction leads to cleavage and functionalization of coordinated dinitrogen. Proc. Natl Acad. Sci. USA 103, 17094–17098 (2006)
Nishibayashi, Y. Recent progress in transition-metal-catalyzed reduction of molecular dinitrogen under ambient reaction conditions. Inorg. Chem. 54, 9234–9247 (2015)
Haber, F. Verfahren zur Herstellung von Ammoniak durch katalytische Vereinigung von Stickstoff und Wasserstoff, zweckmäßig unter hohem Druch. German patent DE 229126 (1909)
Cloke, G. F. N. & Hitchcock, P. B. Reversible binding and reduction of dinitrogen by a uranium(III) pentalene complex. J. Am. Chem. Soc. 124, 9352–9353 (2002)
Odom, A. L., Arnold, P. L. & Cummins, C. C. Heterodinuclear uranium/molybdenum dinitrogen complexes. J. Am. Chem. Soc. 120, 5836–5837 (1998)
Mansell, S. M., Kaltsoyannis, N. & Arnold, P. L. Small molecule activation by uranium tris(aryloxides): experimental and computational studies of binding of N2, coupling of CO, and deoxygenation insertion of CO2 under ambient conditions. J. Am. Chem. Soc. 133, 9036–9051 (2011)
Evans, W. J., Kozimor, S. A. & Ziller, J. W. A monometallic f element complex of dinitrogen: (C5Me5)3U (η1-N2). J. Am. Chem. Soc. 125, 14264–14265 (2003)
Roussel, P. & Scott, P. Complex of dinitrogen with trivalent uranium. J. Am. Chem. Soc. 120, 1070–1071 (1998)
Camp, C., Pecaut, J. & Mazzanti, M. Tuning uranium-nitrogen multiple bond formation with ancillary siloxide ligands. J. Am. Chem. Soc. 135, 12101–12111 (2013)
Chatelain, L., Scopelliti, R. & Mazzanti, M. Synthesis and structure of nitride-bridged uranium(III) complexes. J. Am. Chem. Soc. 138, 1784–1787 (2016)
Spencer, L. P. et al. Cation-cation interactions, magnetic communication, and reactivity of the pentavalent uranium ion [U(NtBu)2]+. Angew. Chem. Int. Edn Engl. 48, 3795–3798 (2009)
Grubel, K., Brennessel, W. W., Mercado, B. Q. & Holland, P. L. Alkali metal control over N-N cleavage in iron complexes. J. Am. Chem. Soc. 136, 16807–16816 (2014)
Tsui, E. Y., Tran, R., Yano, J. & Agapie, T. Redox-inactive metals modulate the reduction potential in heterometallic manganese-oxido clusters. Nat. Chem. 5, 293–299 (2013)
Falcone, M., Kefalidis, C. E., Scopelliti, R., Maron, L. & Mazzanti, M. Facile CO cleavage by a multimetallic CsU2 nitride complex. Angew. Chem. Int. Edn Engl. 55, 12290–12294 (2016)
Cleaves, P. A. et al. Two-electron reductive carbonylation of terminal uranium(V) and uranium(VI) nitrides to cyanate by carbon monoxide. Angew. Chem. Int. Edn Engl. 53, 10412–10415 (2014)
Knobloch, D. J., Lobkovsky, E. & Chirik, P. J. Carbon monoxide-induced dinitrogen cleavage with group 4 metallocenes: reaction scope and coupling to N-H bond formation and CO deoxygenation. J. Am. Chem. Soc. 132, 10553–10564 (2010)
Duisenberg, A. J. M., Kroon-Batenburg, L. M. J. & Schreurs, A. M. M. An intensity evaluation method: EVAL-14. J. Appl. Crystallogr. 36, 220–229 (2003)
Blessing, R. H. An empirical correction for absorption anisotropy. Acta Crystallogr. A 51, 33–38 (1995)
CrysAlis PRO (Rigaku Oxford Diffraction Ltd, Yarnton, Oxfordshire, UK, 2015)
Sheldrick, G. M. A short history of SHELX. Acta Crystallogr. A 64, 112–122 (2008)
Sheldrick, G. M. Crystal structure refinement with SHELXL. Acta Crystallogr. A 71, 3–8 (2015)
Spek, A. L. PLATON SQUEEZE: a tool for the calculation of the disordered solvent contribution to the calculated structure factors. Acta Crystallogr. C 71, 9–18 (2015)
Kindra, D. R. & Evans, W. J. Magnetic susceptibility of uranium complexes. Chem. Rev. 114, 8865–8882 (2014)
Stoll, S. & Schweiger, A. EasySpin, a comprehensive software package for spectral simulation and analysis in EPR. J. Magn. Reson. 178, 42–55 (2006)
Cooper, O. et al. Multimetallic cooperativity in uranium-mediated CO2 activation. J. Am. Chem. Soc. 136, 6716–6723 (2014)
Acknowledgements
We acknowledge support from the Swiss National Science Foundation (200021-157158 and 200021-162430) and from the Ecole Polytechnique Fédérale de Lausanne (EPFL). We thank E. Solari for carrying out the elemental analyses, and D. Kubicky (of the L. Emsley group) for assistance with EPR measurements, M. Prevot (of the K. Sivula group) for assistance with Raman and B. Rozmyslowicz (of the J. Luterbacher group) for assistance with IR and GC-MS measurements. We thank F. F. Tirani for her contribution to the X-ray single crystal structure analyses.
Author information
Authors and Affiliations
Contributions
M.F. carried out the synthetic experiments and analysed the experimental data; L.C. carried out preliminary synthetic experiments, including the isolation of the dinitrogen complex. R.S. performed the X-ray single crystal structure analyses; I.Z. carried out and analysed the magnetic measurements; M.M. and M.F. wrote the manuscript. M.M. originated the central idea, coordinated the work and analysed the experimental data.
Corresponding author
Ethics declarations
Competing interests
The authors declare no competing financial interests.
Additional information
Publisher's note: Springer Nature remains neutral with regard to jurisdictional claims in published maps and institutional affiliations.
Extended data figures and tables
Extended Data Figure 1 EPR and magnetic data.
a, X-band (9.40 GHz) EPR spectrum of 2 in toluene/hexane glass at 10 K (red line, experiment; black line, fit to two 5f1 ions). b, Plot of measured magnetic susceptibility χM for 2 versus temperature. The measurements were repeated twice.
Extended Data Figure 2 Protonation of complex 2.
1H NMR spectra (400 MHz, THF-d8, 298 K) for the successive addition of 1 equiv. PyHCl (up to 7 equiv.) to 2. Bottom trace, before addition of PyHCl. Symbols on peaks show assignments (see key at top); f1, chemical shift.
Extended Data Figure 3 Formation of ammonia from the addition of H+ to 2 and to 15N-2.
Shown are 1H NMR spectra (400 MHz, DMSO-d6, 298 K) of: a, the white precipitate formed after addition of excess PyHCl (20 equiv.) to 2; b, the white precipitate formed after addition of excess PyHCl (20 equiv.) to 15N-2; c, NH4Cl formed after addition of HCl(Et2O) to 2 (2 equiv. of dimethyl sulfone added for quantitative determination). In each panel, symbols on peaks show assignments (see key at top).
Extended Data Figure 4 Hydrogenation of 2 and formation of ammonia from the addition of H2/H+ to 2 and to 15N-2.
a, 1H NMR spectra (400 MHz, C7D8, 298 K) of the crude reaction mixture at different times after reaction of 2 with H2 (1 atm) at room temperature. Bottom trace, immediately after H2 addition. b, c, Quantitative 1H NMR spectra (400 MHz, DMSO-d6, 298 K) of: b, NH4Cl formed after addition of HCl(Et2O) to the residual solid of reaction between 2 and H2 (2 equiv. of dimethyl sulfone added); c, 15NH4Cl and NH4Cl formed in 2:1 ratio after addition of HCl(Et2O) to the residual solid from the reaction between complex 15N-2 and H2 (1 equiv. of dimethyl sulfone added). In each panel, symbols on peaks show assignments (see key at top).
Extended Data Figure 5 Summary of 1H NMR and 13C NMR data for 5.
a, 1H NMR spectrum (400 MHz, C6D6, 298 K) of the crude mixture 1 h after addition of 10 equiv. of CO to 2 to afford 5. b, 1H NMR spectrum (400 MHz, C6D6, 298 K) of crystals of 5. c, 13C NMR spectrum (100.6 MHz, D2O, 298 K) of the crude mixture after addition of 10 equiv. of CO to 2, showing the presence of 13C14N− and O13C15N− in a 1:2 ratio; inset shows the doublet nature of the peak assigned to O13C15N−. In each panel, symbols on peaks show assignments (see key at top).
Extended Data Figure 6 Summary of 1H NMR and 13C NMR data for 6.
a, 1H NMR spectrum (400 MHz, C7D8, 298 K) of crystals of 6. b, 13C NMR spectrum (400 MHz, C7D8, 298 K) of crystals of 6. c, 13C NMR spectrum (400 MHz, D2O (DMSO as reference), 298 K) of the crude reaction mixture after addition of 10 equiv. of CO to complex 3. d, Mass spectrum of the gas evolved during the reaction of 15N-3 with CO, showing formation of 14N15N and 15N2 with m/z = 29 and 30, respectively. The peak at m/z = 28 arises from 14N2 and CO. 14N15N and 14N2 arise from nitride/N2 scrambling during the synthesis of 3. The inset details the measured abundance (intensity) for each peak. In a–c, symbols on peaks show assignments (see key at top).
Extended Data Figure 7 Summary of 1H NMR data for 1, 2 and 3.
a, 1H NMR spectra (400 MHz, THF-d8, 298 K) of [Cs{[U(OSi(OtBu)3)3]2(μ-N)}] before (bottom) and after (top) addition of excess KC8 to yield to 1. b, 1H NMR spectra (400 MHz, C7D8, 298 K) of the crude mixture before (bottom) and after (top) addition of N2 to complex 1. c, 1H NMR spectrum (400 MHz, THF-d8, 298 K) of crystals of 2. d, 1H NMR spectrum (400 MHz, C7D8, 298 K) of crystals of complex 3. In each panel, symbols on peaks show assignments (see key at top).
Extended Data Figure 8 Additional reactivity of 3 and 6.
a, 1H NMR spectra (400 MHz, C7D8, 298 K) of the crude reaction mixture after reaction of 3 with H2 (1 atm) at room temperature for 1 week leading to a new product (top; bottom, immediately after introduction of H2). b, 1H NMR spectrum (400 MHz, C7D8, 298 K) of the crude reaction mixture after addition of 100 equiv. of CO to 3. The chemical shift of the only product (labelled with a blue dot) is 1.08 p.p.m., very close to the chemical shift of complex 5. c, 1H NMR spectrum (400 MHz, DMSO-d6, 298 K) of the white precipitate formed after addition of excess PyHCl (20 equiv.) to 15N-6. In each panel, symbols on peaks show assignments (see key at top).
Rights and permissions
About this article
Cite this article
Falcone, M., Chatelain, L., Scopelliti, R. et al. Nitrogen reduction and functionalization by a multimetallic uranium nitride complex. Nature 547, 332–335 (2017). https://doi.org/10.1038/nature23279
Received:
Accepted:
Published:
Issue Date:
DOI: https://doi.org/10.1038/nature23279
This article is cited by
-
N2 cleavage by silylene and formation of H2Si(μ-N)2SiH2
Nature Communications (2024)
-
Hydroamination of alkenes with dinitrogen and titanium polyhydrides
Nature (2024)
-
Advances in electrochemical transformation of N2 using molecular catalysts
Science China Chemistry (2023)
-
Ammonia formation revisited
Nature Chemistry (2022)
-
A thiolate-bridged FeIVFeIV μ-nitrido complex and its hydrogenation reactivity toward ammonia formation
Nature Chemistry (2022)